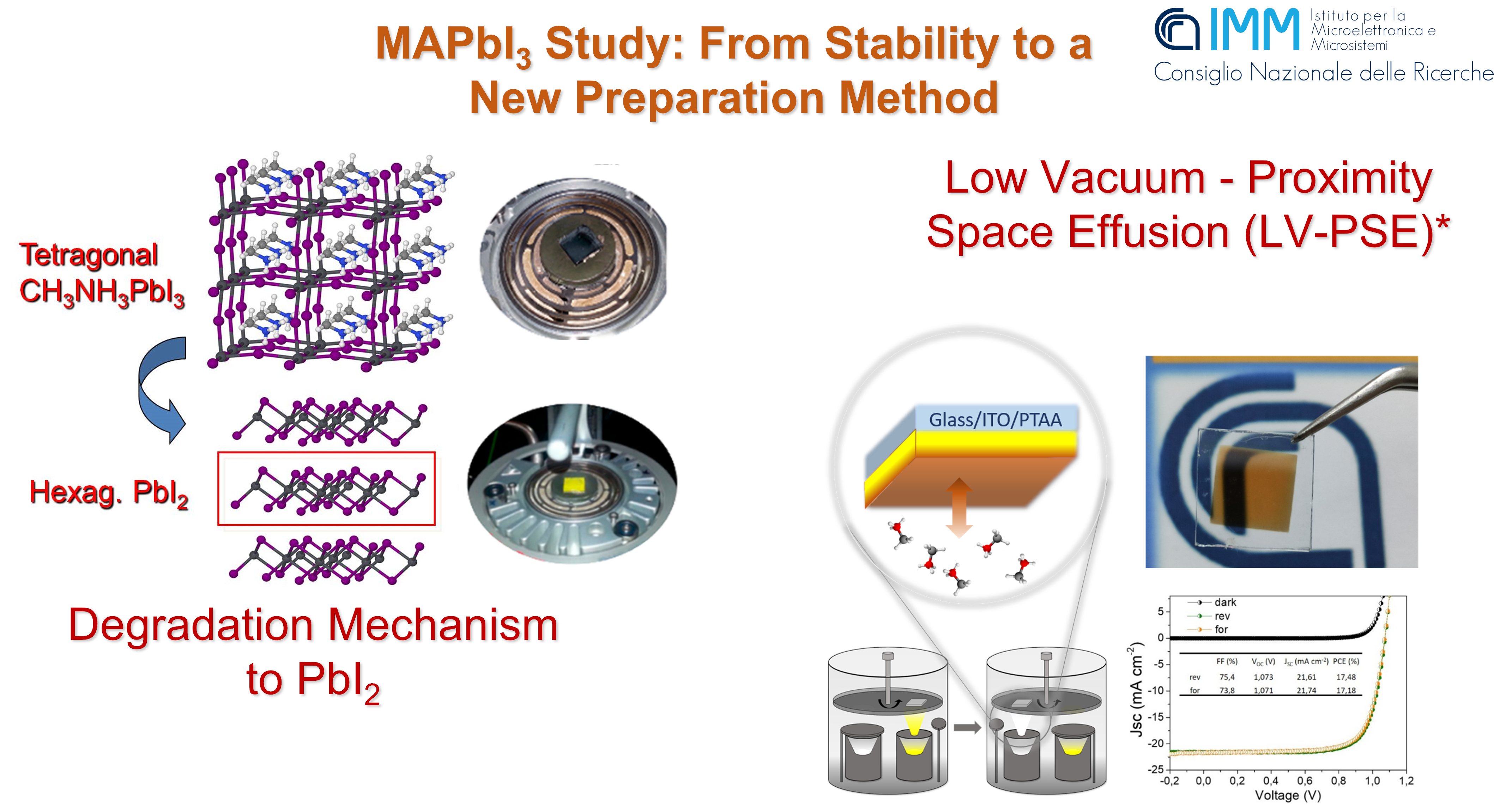
Hybrid Organic-Inorganic Perovskites are worldwide one of the most investigated materials today, due to their unique properties impacting various fields as Photovoltaics, [1-4] Light Emission Devices(LEDs) [5,6] and Photodetectors [7-9]. In PV, thanks to the wide absorption range and excellent charge carrier lifetime and diffusion [10] of the material, Perovskite Solar Cells (PSCs) were able to reach the exceptional goal of 25.7% [11] just ten years after the first publication of Miyasaka et al.[12] in 2009. All their exceptional properties, deriving from the possibility of preparing high quality films in terms of crystallinity, thickness and low defect density, are strictly related [13-15]. The first used and most famous hybrid perovskite is Methylammonium lead iodide (MAPbI3). We firstly focused our attention on stability and investigation of degradation mechanism of MAPbI3 and we provided a semi-empirical model based on in situ degradation measurements to predict the durability of hybrid perovskite materials under simulated thermal operation condition. In the model, the degradation path of MAPbI3 layers is proved to follow an Arrhenius-type law [16]. Then we focused on stabilization and on avoiding degradation of MAPbI3 showing the role of nitrogen molecule general paradigm is drawn that reconsiders N2 not simply being an inert species but rather an effective healing gas molecule if entering a methylammonium lead iodide (MAPbI3) layer [17]. Then we developed an innovative vacuum deposition method to prepare CH3NH3PbI3 (MAPbI3) thin film for semitransparent perovskite solar cells. The method applies a two steps Low-Vacuum Proximity-Space-Effusion (LV-PSE) under low vacuum conditions to produce high-quality thin layers of phase-pure MAPbI3. Using 150 nm-thick films we were able to achieve 14.4% mean efficiency and a best stabilized efficiency value of 16.5% [18].
[1] J. Burschka, N. Pellet, S.-J. Moon, R. Humphry-Baker, P. Gao, M. K. Nazeeruddin and M. Grätzel, Nature, 2013, 499, 316–9.
[2] N. J. Jeon, J. H. Noh, W. S. Yang, Y. C. Kim, S. Ryu, J. Seo and S. Il Seok, Nature, 2015, 517, 476–480.
[3] A. K. Jena, A. Kulkarni, and T. Miyasaka,Chem. Rev., 2019, 119, 3036-3103
[4] W. Huang, J. S. Manser, P. V. Kamat and S. Ptasinska, Chem. Mater., 2016, 28, 1, 303–311.
[5] M. Zhang, F. Yuan, W. Zhao, B. Jiao, C. Ran, W. Zhang and Z. Wu, Org. Electron. Physics, Mater. Appl., 2018, 60, 57–63.
[6] V. Adinolfi, W. Peng, G. Walters, O. M. Bakr and E. H. Sargent, Adv. Mater., 2018, 30, 1–13.
[7] Zhou and J. Huang, Adv. Sci., 2018, 5. 1700256
[8] N. Patel, S. Dias and S. B. Krupanidhi, J. Electron. Mater., 2018, 47, 2306–2315
[9] H. W. Chen, N. Sakai, A. K. Jena, Y. Sanehira, M. Ikegami, K.-C. Ho and T. Miyasaka, J. Phys. Chem. Lett., 2015, 6, 1773–1779
[10] S. D. Stranks, G. E. Eperon, G. Grancini, C. Menelaou, M. J. P. Alcocer, T. Leijtens, L. M. Herz, A. Petrozza and H. J. Snaith, Science, 2013, 342, 341–344
[11] https://www.nrel.gov/pv/assets/pdfs/best-research-cell-efficiencies.2020...
[12] A. Kojima, K. Teshima, Y. Shirai and T. Miyasaka, J. Am. Chem. Soc., 2009, 131, 6050–1
[13] Y. Zhao and K. Zhu, J. Phys. Chem. Lett., 2014, 5, 4175–4186.
[14] W. S. Yang, J. H. Noh, N. J. Jeon, Y. C. Kim, S. Ryu, J. Seo and S. Il Seok, Science, 2015, 348,1234-1237
[15] A. Ng, Z. Ren, Q. Shen, S. H. Cheung, H. C. Gokkaya, S. K. So, A. B. Djurišić, Y. Wan, X. Wu and C. Surya, ACS Appl. Mater. Interfaces, 2016, 8, 32805–32814
[16] E. Smecca, Y. Numata, I. Deretzis, G. Pellegrino, S. Boninelli, T. Miyasaka, A. La Magna and A. Alberti. Phys. Chem. Chem. Phys., 2016, 18, 13413
[17] A Alberti, I. Deretzis, G. Mannino, E. Smecca, F. Giannazzo, A. Listorti, S. Colella, S. Masi, A. La Magna. Adv. Energy Mater. 2019, 1803450.
[18] E. Smecca, V. Valenzano, S. Valastro, I. Deretzis, G. Mannino, G. Malandrino, G. Accorsi, S. Colella, A. Rizzo, A. La Magna, A. Listorti, A. Alberti. J. Mater. Chem. A, 2021, 9, 16456-16469